Sodium-ion batteries (SIBs) are gaining attention as one of the most viable next-generation alternatives to lithium-ion batteries (LIBs) due to the abundance of material resources and low cost.
Rechargeable lithium-ion batteries (LIBs) have unmatched energy density and versatility. From electronic gadgets to electric vehicles (EVs), they are widely used, and their popularity is increasing day by day as they provide fast-charging rates and long life cycle. However, their ever-increasing application, global demand, and rising cost due to the declining availability of lithium and other raw materials such as cobalt, copper, and nickel are causing unprecedented pressure on the supply chain. Sodium-ion batteries (SIBs) are emerging as the most promising technology for replacing LIBs, as they share the same fundamental working principle, cell construction, power delivery characteristics, and wide application due to their low charging time, greater life cycle, and low cost. SIBs use raw materials that are abundant, cheaper, have a reduced impact on the environment, and provide better safety during operation and transportation. Additionally, they can be discharged completely to zero volts, causing no risk of thermal runaway, and perform effectively at temperatures ranging from as low as minus 20 degrees Celsius to as high as plus 65 degrees Celsius. Therefore, sodium-ion batteries (SIBs) are emerging as a viable technology on the cusp of commercialisation. Presently, SIBs are considered most suited for applications such as short-range electric vehicles and large-scale energy storage systems. They also have the potential to store renewable energy generated from sources like solar and wind power and can serve as a more cost-effective choice for electric vehicles.
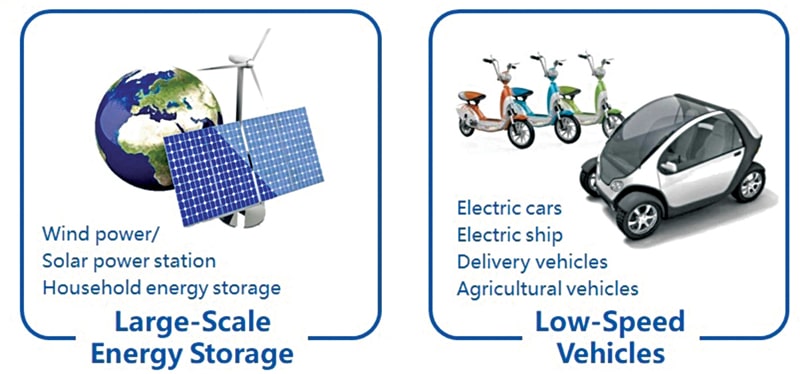
Evolution of the sodium-ion batteries technology
Although SIBs have been studied since the early 1970s, their development somewhat slowed down due to the increasing popularity and high demand for LIBs. However, research was revived in earnest during the late 1990s as the prices of LIBs started to increase due to rising raw material costs and supply chain issues. Additionally, serious concerns were being raised about the adverse impact on the environment and increasing safety issues during operation. The first sodium-ion cell using hard carbon was demonstrated way back in 2003, showing an average voltage of 3.7V during discharge. The development gained momentum only during the last decade as SIBs were found to be the most promising alternative to LIBs. Efforts are now underway to establish scalable production technology capable of meeting the commercial targets of a lifetime cost less than £0.1/kWh, deep discharge cycle life greater than 10,000 cycles, capital expenditure less than £250 (usable kWh installed), volumetric energy density greater than 20kWh per cubic metre, and lifetime over 10 years. While initially, Japanese scientists made crucial advances, Chinese companies are now leading in commercialising the technology. It is expected that in the next two years, China will have nearly 95 percent of the world’s capacity.
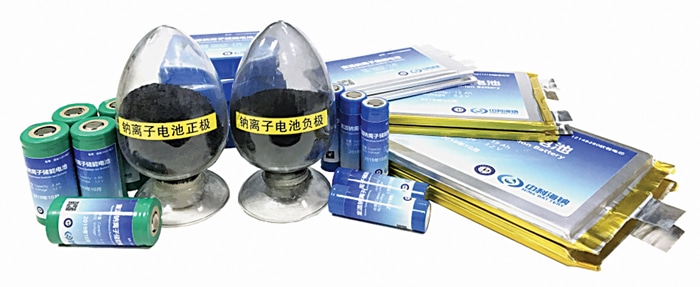
Recently, China-based HiNa Battery Technology demonstrated an electric test car using a 140Wh/kg SIB. The world’s biggest battery manufacturer, CATL, has announced that it will begin mass production in 2023. However, commercially available EVs using sodium-ion batteries are not yet available.
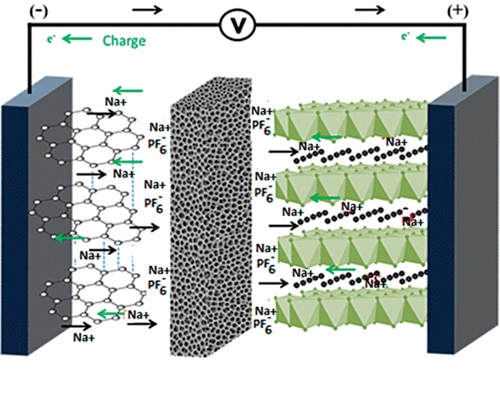