It is much easier to find our way across a neat and well-ordered store than a messy and disorganised one. Electronic conduction shows a similar preference; ordered, crystalline materials make much better conductors than disordered, amorphous materials. Single-crystal conductors show much lower resistance to electric current than their polycrystalline or amorphous forms. This is the reason why almost all electronic devices are made from monocrystalline semiconductors.
The difference in resistivity between crystalline and amorphous forms of the same conductor can be very big. This should not come as a surprise because, after all, resistivity is a physical property that can vary over an enormous range with the ratio of resistivity between good and bad conductors easily exceeding 1018, at a given temperature.
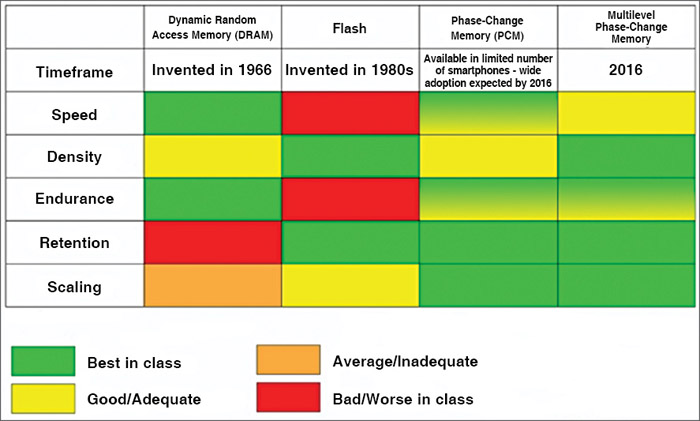
The remarkable contrast between the resistivities of ordered and disordered phases of certain conducting materials is the basis of an emerging memory technology called phase-change memory (PCM), which promises to revolutionise the digital system design in the near future. It has taken many years and sustained efforts of many individuals and companies for phase-change technology to leave the confines of research laboratories and become a commercial reality. By all indications, this is a revolutionary new device with much promise for future applications.
The science of PCM material
Elements belonging to group VI of the periodic table are called chalcogens. These elements can combine with other electropositive elements to form semi-metallic compounds called ‘chalcogenides.’ Some of these compounds have a remarkable property of being able to exist in either a crystalline or an amorphous state with very small transition energy to go from one state to the other.
If such a material is melted and then cooled quickly, its constituent atoms do not get the time to settle in an ordered crystal lattice, producing a disordered metallic glass phase having high resistivity. However, if the molten material is cooled more slowly, a low-resistivity crystalline phase forms.
What is astounding is that the transition from one phase to another can be affected in just a few tens of nanoseconds (ns). The high phase-transition speed and the huge difference in resistivity between the crystalline and amorphous phases in a phase-change chalcogenide can be used to store the binary data.
Phase-change memories use a material called ‘germanium antimony telluride’ (Ge2Sb2Te5), abbreviated as GST. Its low-resistivity crystalline phase can be used to represent binary ‘1’ (set state), whereas its high-resistivity amorphous phase can be used to code for binary ‘0’ (reset state). One can change the logic state by controlled heating of the phase-change material—rapidly heating and cooling the molten material quenches the material to amorphous phase, whereas somewhat slower heating anneals the material to its crystalline state. In a memory device, heating can be performed by tiny heaters embedded within each memory cell.
The origin
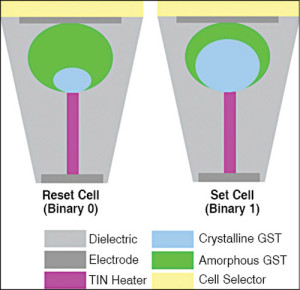
Stanford R. Ovshinsky was the first person to consider using resistive changes in phase-change materials as the basis for storing digital data. He patented this idea in the mid sixties and published a paper on it in 1968, when he owned a company, named Energy Conversion Devices Inc. Ovshinsky later founded another company called Ovonyx to commercialise the technology for memory devices.
For many years the development failed to get much traction because of the unavailability of sufficient funds. During the 1990s, a joint venture between Intel and STMicroelectronics acquired the technology rights from Ovonyx and formed a company called Numonyx in 2008, to further develop the PCM technology.
Numonyx quickly brought out a prototype 256Mb memory device fabricated with 90nm technology. Soon after, Samsung demonstrated a 512Mb PCM random-access memory (PRAM) chip made with an 58nm process technology. Numonyx had difficulty surpassing this offer from its South Korean rival so it subsequently focused on stacking several 128Mb chips to produce higher-capacity devices, although none was commercialised. By 2010, Numonyx was sold to the pure memory manufacturer Micron Technologies of Boise, Idaho, and now Micron and Samsung are the main contenders in the PCM race.
Competing with other technologies
As both the crystalline and amorphous phases are stable, PCMs are non-volatile, i.e., the memory retains its contents even if the power is switched off. It is very interesting to compare the attributes of PCM with Flash memory—the most widespread non-volatile memory technology at this time. Fig. 1 lists pertinent features of the two technologies (also included are dynamic RAM attributes, for reference).
The main drawback of the contemporary Flash memories is their slow access time, which relegates them to be used only as storage devices. They also require erasing of a whole block before even one bit can be changed—a process that needs several milliseconds.
PCMs, on the other hand, feature write speed of around 100 ns and read speed of around 20 ns, which make them blazingly fast. This is all due to the exceptionally fast speed with which the crystalline-amorphous phase change can be affected in materials such as GST.
Flash memories can have very high densities because each memory cell consists of a floating-gate memory transistor tightly coupled with a cell selector transistor, with their combination taking up very little silicon area. The actual phase-change memory cells in a PCM device are very small but the cell-selection transistor required to select each memory cell (bit location) is relatively large. This somewhat limits the achievable integration density, but rapid progress is being made to overcome this limitation and to produce high bit-count PCM chips.
The maximum number of read-write cycles possible with Flash memory is quite limited. Most commercial-grade Flash memory chips are guaranteed for at most 5000 memory cycles because of the wear and tear on memory transistors due to the high programming voltages used.
PCM wins outright here because the crystalline-amorphous phase changes cause no material degradation. PCM chips are guaranteed for at least one million read-write cycles, which makes them suitable for long-life embedded applications with frequent data changes. Such applications can also benefit from the high data retention time of PCM devices, which exceeds 10 years at ambient temperatures.
Finally, Flash memories are going to run into a fundamental scaling limitation once their minimum feature size gets below 22 nm. It will be hard to scale them down further because memory transistors of such small dimensions hold only a few hundred electrons to signal the state as ‘0’ or ‘1.’ Random thermal fluctuations can flip the logic state, making the memory increasingly unreliable.
PCM devices, in comparison, can be scaled down to five nanometres in minimum feature dimension without any adverse effects because their structure is much simpler and the phases are immune to random thermal fluctuations. This feature has been much discussed in the technical circles and there is some agreement that PCM is the successor to Flash technology—gradually overtaking the latter as the minimum process technology dimension shrinks below 22 nm.
Yet another advantage of PCM stems from the fact that data is stored as a stable structural phase rather than as the presence or absence of charge carriers, as is the case with other current non-volatile memory technologies. This means that PCM is not affected by ionising radiation and is thus useful for military and aerospace applications. This prospect has made defence companies like BAE Systems and Lockheed Martin take serious interest in this memory technology.
Structure and function
Tiny memory cells made from very thin GST films and a titanium-nitride (TiN) heater are fabricated as a memory array on top of complementary metal-oxide semiconductor (CMOS) circuitry for selecting, reading and writing individual cells. There is a cell selector device placed either above or below each memory cell.
The cell selector, which can be either a p-n-junction diode, a bipolar or field-effect transistor, or some other suitable device, ensures that only the addressed memory cell is selected. The selector device also provides heating current to the cell for changing its state, as required during a write operation. The cell selector must be able to provide the needed current and must have low leakage itself to reduce the overall power consumption.
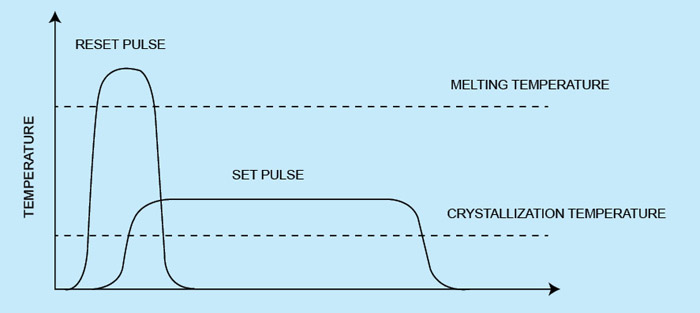
Fig. 2 shows the structure of a PCM cell in set and reset states. It is advantageous to reduce the contact area between the TiN heater and the phase-change material. This increases the rate of heating by reducing thermal time constants and also the value of heating current needed to change the material’s phase.