All electronic devices and circuitry generate excess heat and, thus, require thermal management to improve reliability and prevent premature failure.
Efficiency of an electronic device is inversely proportional to its temperature. A rise in temperature leads to a subsequent drop in performance. High-performance electronic components generate heat loads that create excessive junction temperatures, compromise component lifetimes and can lead to the premature failure of electronic devices.
As a result, there is a need to lower the temperature of and/or remove heat from electronic devices. This is a challenge often faced by the mobile device industry. One of the most challenging task is the ability to keep components cool, because overheating causes significant reduction in the operating life of a device and leads to device failure.
As electronic devices get smaller, engineers and designers are faced with the growing challenge of keeping up with the need to optimise processing speed within a shrinking form factor. Faster processors necessitate increased power consumption, which generates heat.
A smaller form factor necessitates greater miniaturisation of the implements used to disperse that heat. These considerations are forcing engineers and designers to think in terms of systemic solutions in which every consideration in a device’s power equation is examined for greatest optimisation.
In general, requirements of the power equation demand that heat dissipation must be proportional to the power dissipation of a given device. Power dissipation is the amount of electricity wasted by a device (power dissipation is dependent on capacitance of logic elements, operating voltage swing and operating frequency).
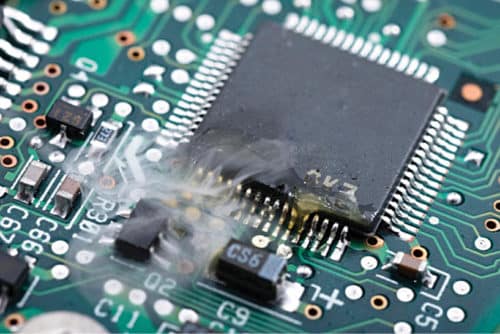
Three major challenges to the problem of managing power dissipation in today’s small-footprint electronic devices are dense printed circuit boards (PCBs), increased heat density in integrated circuit (IC) chips, size of devices and mobility desired by users.
Most electronic components are low power and produce negligible amounts of heat in their operation. Some devices (power transistors, CPUs and power diodes), however, produce a significant amount of heat. Measures may be necessary to prolong their working life and increase reliability.
Let us consider a heat-producing electronic component in isolation. During operation, its temperature will rise until the heat produced within the device becomes equal to the heat lost to the surroundings, and the device has reached equilibrium.
Heat is generated due to the resistance offered by the material for the flow of electrons within it. It is given by the famous Joule’s law of heating due to current flow as H=I2Rt (where, H is heat generated, I is current flowing, R is resistance offered by the material and t is time for which current is allowed to flow in the material). Resistance offered by the material for current flow is the main factor responsible for heat generation (if I and t are taken to be controlled). Lesser the resistance, greater the conductivity, and, thus, low heat generation. Hence, there are simultaneous efforts going on towards the research on high-conductivity materials.
Thermal management
Managing the heat generated by components has always been an important element of electronic design. It is even truer today, as power densities continue to increase and individual components such as microprocessors continue to draw more power to meet the demanding functional and computational requirements of current high-powered applications.
To ensure proper performance and reliability, excess heat must be transported away from critical components and system hot spots, and then dissipated into the ambient environment. Engineers can use intelligent thermal designs to prevent heat-related failures, increase the life expectancy of the systems and reduce emitted noise, energy consumption, cost and time-to-market.
Heat conduction occurs via motion of electrons and phonons. While the bulk properties of the materials determine the fundamental transport and scattering of these heat carriers, properties of interfaces and finite dimensionality are key issues in applications.
Three driving forces are placing stringent demands on materials for thermal management.
First, the drive to improve speed motivates circuit designers to compress the core of the microprocessor, which contains the region of the most electrical activity, to ever smaller sizes. This gives higher rates of heat generation per unit area. As a result, temperature drop caused by solid conduction within the chip and the heat spreader is predicted to become comparable to the maximum allowed temperature drop. The problem is compounded by exploratory three-dimensional circuits, which increase connectivity but also increase the difficulties associated with heat removal.
Second, temperature rise in interconnects between transistors is growing. This is due to increases in the number of metal layers and increasing interconnect current densities and aspect ratios. The problem is made worse by planned transition to interconnect passivation with lower dielectric constants than silicon dioxide (SiO2).
Finally, there is the increasing temperature rise within contemporary and planned transistor technologies. Decreasing channel dimensions increases power density and electron-phonon non-equilibrium within devices. Novel device geometries (silicon-non-insulator, nano-pillars and fin-shaped field-effect transistors) offer greater thermal resistances to the bulk substrate.
These trends pose important challenges and opportunities for fundamental research in materials and solid-state physics.
Cooling of electronics
The rate of loss of heat from a hot object is governed approximately by Newton’s Law of Cooling. The law states that the rate of loss of heat is proportional to the temperature difference between the body and the surroundings. As temperature of the component rises, heat loss increases. When heat loss per second equates the heat produced per second within the component, the device achieves its equilibrium temperature. This temperature may be high enough to significantly shorten the life of the component, or even cause the device to fail. It is in such cases that thermal management measures need to be taken.
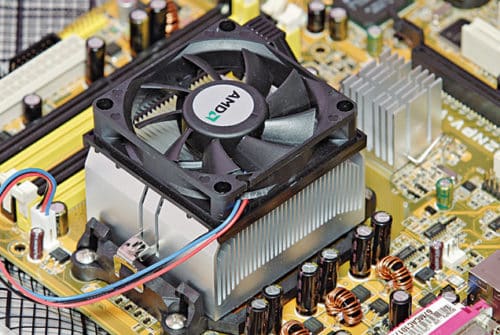
The same considerations can be applied to a complete circuit or device that incorporates heat-producing individual components. Rate of loss of heat is higher in a forced draught than still air. One way of controlling the temperature of a device or circuit is to incorporate a fan, or fans, to increase air flow. Even ensuring adequate general ventilation results in a lower operating temperature than if the circuit is in a confined space with no ventilation slots.
One point that can be overlooked is that, reduced atmospheric density at high altitudes leads to less effective heat transfer to the surroundings and consequent higher device operating temperatures. There are several techniques for cooling, including various styles of heat-sinks, thermoelectric coolers, forced air systems and fans, heat pipes and so on. In cases of extreme low environmental temperatures, it may be necessary to heat the electronic components to achieve satisfactory operation.
Heat-sinks
Heat is lost from a component to its surroundings at the surface of the component. Rate of loss of heat increases with the surface area of the component. A small device producing 10 watts reaches a higher temperature than a similar powered device with a larger surface area.
One way of limiting the operating temperature is to artificially increase the surface area. This is done by attaching a metal heat-sink to the device. A heat-sink can be made by stamping, extrusion or casting. It is generally fabricated from copper or aluminium or their alloys—the heat-sink needs to be a good conductor of heat.
Often, heat-sinks have a finned structure to maximise the available area for heat dissipation to the surroundings. Where heat-sinks are being used, these are more effective if the whole unit is well ventilated or, even better, forced air flow is applied by use of one or more fans.
It is impossible to make heat-sinks or components with perfectly flat mating surfaces, so when the two come into contact, high spots contact one another and there is a small air gap between the two over a large part of the surface.
Air is a poor conductor of heat and so the interface provides a thermal barrier. This limits the efficiency of heat loss from the device. It is to overcome this effect that heat transfer compounds are used.
More recently, synthetic diamond cooling-sinks are being researched to provide better cooling. Also, some heat-sinks are constructed of multiple materials with desirable characteristics, such as phase-change materials, which can store a great deal of energy due to their heat of fusion.
Heat spreaders
Another often-used cooling device is a heat spreader. It is a thermally conductive metal plate or foil used to move concentrated heat and distribute it over a wider area. It is normally used as an intermediate thermal interface between a heat source and a secondary heat exchanger, such as a heat-sink.
A heat spreader is often applied as a backing plate to PCBs containing heat-generating components. Thermal vias are placed in the PCB, which act as thermal conduits between each component package and the heat spreader to improve heat flow. Some PCB technologies eliminate the need for an external plate by integrating a metal core layer directly into the board that allows the PCB itself to act as a heat spreader.
Heat pipes
An extremely efficient method of thermal conduction is provided by heat pipes. These devices consist of a sealed, hollow tube containing a liquid or coolant. One end of the tube is attached to a heat source and the other to a secondary heat exchanger, such as a heat-sink. Heat generated from a component boils the liquid at one end of the pipe, which then travels to the cool end. The vapour condenses (giving off latent heat) and returns back to the heated end through capillary action or gravity. Heat pipes are typically made of a thermally conductive metal, which can be flat or bent into complex shapes. This makes these very suitable for space-limited board designs.
Thermal interface materials
An important means of optimising heat transfer between a heat source and a cooling device (or between two adjacent cooling devices) is the use of thermal interface materials. These are thermally conductive, pre-manufactured materials that can be applied in a variety of forms. These include thermal pads, adhesives, gels and grease. These are designed to conform to surface irregularities and imperfections, which allow these to fill in air gaps between contact surfaces. This ensures that maximum available surface area is being used for heat transfer.
With heat-generating circuitry it may be adequate to ensure thermal control by potting into a metal box, with or without integral or attached cooling fins, using a thermally-conductive potting compound.
Heat transfer compounds are designed to fill the gap between the device and the heat-sink and, thus, reduce the thermal resistance at the boundary between the two. This leads to faster heat loss to the heat-sink and a lower operating temperature for the device.
Heat transfer compounds can be of various types. Electrolubes produce a range of thermally-conductive pastes, which can be applied to displace air from the component/heat-sink interface. These consist of thermally-conductive mineral fillers in a carrier fluid—the fluid may be non-silicone or silicone based.
Silicone-based pastes generally have higher operating temperatures than their non-silicone alternatives. Silicones can cause a problem with some circuitry as these migrate very readily and can cause contamination on, say, relay contacts. It is possible to modify the thermal conductivity of thermally-conductive pastes by increasing the mineral filler content, or changing the types of filler used. These have a higher filler loading and contain a special blend of different fillers to maximise thermal conductivity.
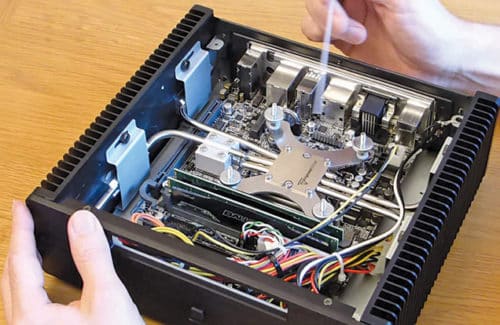
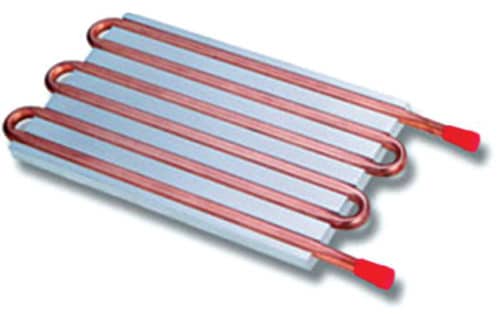
Generally, higher thermally-conductive pastes are higher in viscosity. This can lead to greater difficulty in dispensing. Thermally-conductive pastes remain as pastes. This makes for easier disassembling of components for recovery or repair. It may be desirable in some circumstances to use a thermal transfer material that cures up to a solid. With any thermally-conductive material it is very important to ensure that the interface between the device and the heat-sink is completely filled and all air is displaced.
Cold plate
Placing a conductive thick metal plate, referred to as a cold plate, as a heat transfer interface between a heat source and a cold flowing fluid (or any other heat-sink) may improve cooling performance. In such an arrangement, the heat source is cooled under the thick plate instead of being cooled in direct contact with the cooling fluid. A thick plate can significantly improve the heat transfer between the heat source and the cooling fluid by way of conducting the heat current in an optimal manner.
The two most attractive advantages of this method are no additional pumping power and no extra heat transfer surface area. This is quite different from fins (extended surfaces).
Forced air
The most common method of active cooling is the application of forced air over a heat source. This is accomplished by using a fan or blower to increase the flow of air directly above a heat-generating component or a passive cooling device, such as a heat-sink. The increased air flow moves heated air away at a faster rate. This significantly improves overall heat dissipation.
Fans of different sizes and configurations can be used to control the flow rate, while the flow path can be optimised by their proper placement and orientation. This is especially important in enclosed systems where heated air must be channelled to an exhaust vent. More complex systems utilise electronic fan control to manage both variables based on feedback from localised temperature sensors.
Another type of active heat management is pumped liquid cooling. This closed-loop system consists of a circulating liquid coolant moved by a pump through discrete tubing to remove heat from a heat source.
In electronic cooling, the most common application of this technology is cold plates. A cold plate is a thermally-conductive metal containing internal channels or embedded tubing, which allows liquid coolant flow. It is typically mounted on the back of a PCB containing heat-generating components. The heat source heats up the liquid coolant inside the cold plate. This is pumped to a secondary heat exchanger, where it is cooled. The cooled liquid is then returned to the cold plate, where the heat transfer cycle repeats.
Solid-state heat pumps
These semiconductor devices are often referred to as thermoelectric coolers (TECs). These are thin and compact, and typically placed between a heat source and a heat-sink to facilitate quicker heat dissipation. When a voltage is applied to a TEC, a temperature differential is formed between the two sides of the device. This enables heat transfer to occur through conduction.
While TECs are not very efficient, these still move substantial amounts of heat and have a very long service life. TECs are ideal for applications requiring spot cooling or where sub-ambient temperatures are required.
In addition, if current is reversed on a TEC, heat transfer flow reverses. This essentially turns the device into a heater. This can be ideal for applications where precise temperature control is needed.
Electrostatic fluid acceleration
An electrostatic fluid accelerator (EFA) pumps a fluid, such as air, without any moving parts. Instead of using rotating blades, as in a conventional fan, an EFA uses an electric field to propel electrically-charged air molecules. Because air molecules are normally neutrally charged, the EFA has to create some charged molecules, or ions, first.
Thus, there are three basic steps in the fluid acceleration process: ionising air molecules, using those ions to push many more neutral molecules in a desired direction and then recapturing and neutralising the ions to eliminate any net charge.
The basic principle has been understood for some time but only in recent years has seen developments in the design and manufacture of EFA devices that may allow these to find practical and economical applications, such as in micro-cooling of electronic components.
Role of nanotechnology
For the first time, an international team of scientists led by a researcher at University of California, Riverside has modified the energy spectrum of acoustic phonons—elemental excitations, also referred to as quasi-particles, that spread heat through crystalline materials like a wave—by confining these to nanometre-scale semiconductor structures.
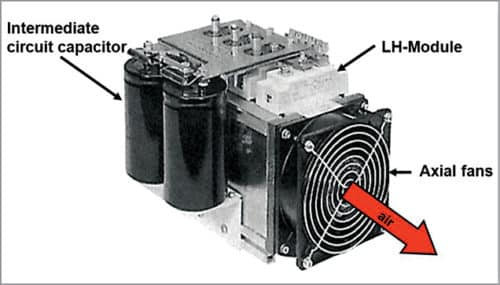
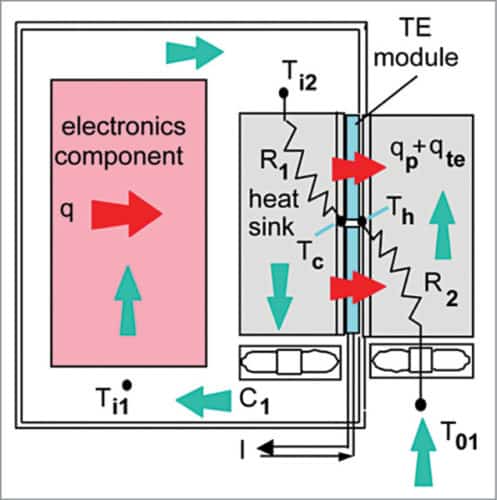
The results have important implications in the thermal management of electronic devices. The team used semiconductor nanowires from gallium arsenide (GaAs), synthesized by researchers in Finland, and an imaging technique called Brillouin-Mandelstam light scattering spectroscopy (BMS) to study the movement of phonons through crystalline nanostructures. By changing the size and the shape of GaAs nanostructures, the researchers were able to alter the energy spectrum, or dispersion, of acoustic phonons.
Controlling phonon dispersion is crucial for improving heat removal from nano-scale electronic devices, which has become a major roadblock in allowing engineers to continue to reduce their size. It can also be used to improve the efficiency of thermoelectric energy generation. In this case, decreasing thermal conductivity by phonons is beneficial for thermoelectric devices.
Dr S.S. Verma is a professor at Department of Physics, Sant Longowal Institute of Engineering and Technology, Sangrur, Punjab