Ultra-low-power techniques are emerging for operation of devices without dependence on batteries. Will such emerging energy harvesting techniques be the key for sustaining low-power devices? Will the devices be feasible for their applications? If so, how?
Most portable electronic devices we use are battery powered. However, innovative solutions for battery-less designs are being constantly developed by researchers and product designers. It is the beginning of a new era, where the latest wireless electronics show the best of both the worlds by being portable and battery-free.
Researchers across the globe have figured out various ways to harvest electricity from ambient environments and power devices. Recent studies have suggested that a significant amount of power can be harnessed from surroundings to power low-power electronic devices like IoT sensors, actuators, etc.
The technologies discussed here enable battery-less solutions and how researchers and design engineers are progressing towards implementing them in an effective way.
Power for small devices
With technologies like Bluetooth Low Energy (BLE) coming into picture, there have been many new advancements in extremely power-saving ICs, sensors, and radio technologies. For designing energy harvesters or alternative storage devices for such low-power systems, understanding power consumption is critical.
The amount of energy a device requires depends on the system performance and the time taken by it to capture and transmit data. Bluetooth Low Energy and similar protocols, such as ZigBee Green Power, are optimised for short frame duration and low transmission power. Such devices are designed to transmit a complete data frame in a few milliseconds.
Sleep mode and deep sleep mode play an important role here. The longer the device stays awake, because of high energy consumption the complexity to make an energy harvester increases for such devices. If a sensor based subsystem requires 3mA at 1V, then the energy budget would be 30µJ per process. The important thing here is that the harvester must be able to supply reliably throughout its entire activity cycle. If this activity interval is between one and ten seconds, the harvester source must operate effectively during that interval.
Majority of IoT devices rely on cellular networks. With vast amounts of data across these networks, the rate of processing is slow and high latency is created. Latency here refers to the time delay from when data is sent from a connected device till it returns to the same device. Low latency is desired for IoT networks, but it comes at a price of high power usage. More energy is needed for faster data transfer. Hence, designers must balance speed and energy consumption.
Energy harvesting techniques
Energy harvesting refers to harnessing small amounts of ambient energy present in environment to power low-power electronic devices. One of the earliest applications was of crystal based radio receiver where power of the received radio signals is used to produce sound, requiring no external power supply.
Nowadays, there are various techniques to harvest this energy from the surrounding environment. These techniques can be easily applied for compact low-power devices where methods like solar power generation are not viable. The power harnessed by these techniques is enough to power small, wireless autonomous devices, like those used in wearable electronics and wireless sensor networks.
Let us have a look at some popular energy harvesting methods and understand how exactly they harvest energy.
Vibrational energy harvesters
If a low-power design demands on-chip energy harvesting solution, then vibrational energy harvesters are perfect for use, especially for wireless nodes. Vibrational energy harvesters derive power from physical vibrations by using micro electromechanical systems (MEMS). It is the most suitable technology to realise IoT-sensing nodes because on a single chip (or a package), this technology enables integrated fabrication of sensors/actuators, electronic circuits for processing information and radio frequency communication, antennas, and energy harvesters.
For using vibrational energy harvesters as a power source, the average power consumption must be tuned around 100µW for a reliable operation. For wireless systems, this tuning can be done by reducing the transmitting duty ratio.
As mentioned, these harvesters take advantage of mechanical energy present in the surroundings. The surrounding mechanical vibrations in the environment have a particular dominant frequency. When the fabricated MEMS oscillator is tuned to this dominant frequency, we can expect to see power generated.
This concept is similar to the impedance-matching concept in which for maximum power transfer, we match the load with the output impedance of the circuit. Such a mechanism is usually referred to as the Velocity-Damped Resonance Generator (VDRG), as the velocity of a physically exciting mass is mechano-electrically coupled to deliver electrical power to the external load.
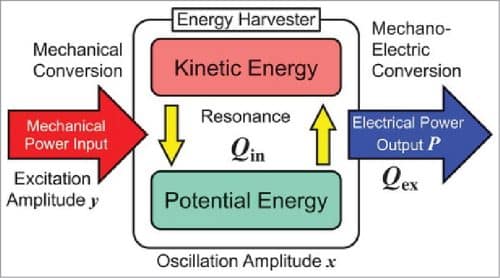
Fig. 1 shows the power conversion mechanism in vibrational energy harvesters. The ‘Qin’ and ‘Qex’ refer to the internal and external quality factors, respectively. Large internal losses are present when Qin is small, since the mechanical resonance is not efficiently excited and there would be a low output if there is insufficient coupling between mechanical and electrical systems when Qex is large. Similar to impedance matching, the maximum power at the output can be obtained if Qin=Qex.
Below are a few examples of energy harvesting devices that include the concept of mechanical energy harvesting.
Electrostatic devices
In the presence of an electric field, when mechanical motion of a conductor occurs, electrostatic current is induced. The electrostatic devices use permanent electric charges or electrets that build the electrostatic fields by trapping electrons or ions in electrical insulator materials. Electrets generate internal and external electric fields and can store charges for a prolonged period of time (say 100 years).
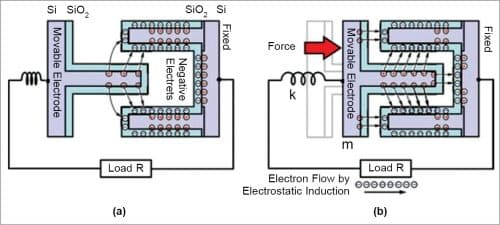
Electromagnetic devices
This electromagnetic harvester follows Faraday’s law that induces an electric field by the changes in magnetic field. The mechanical motion of permanent magnets and coils induces electric power, and their relative motion dictates the amount of electrical power generated.
One of this device’s uniqueness is that even the single-coil and a magnet pair can be operated in two different configurations, depending on the direction of relative displacement.
Piezoelectric devices
Piezoelectric materials are very popular in converting mechanical vibrations into electrical energy. The piezoelectric effect occurs when mechanical strain is applied to piezoelectric materials. These materials get electrically polarised under such conditions in proportion to applied strain. The piezoelectric based energy harvesters have higher energy density for all practical low-power applications.
RF energy harvesters
Radio frequency (RF) is an abundant source, and the concept of harnessing it is quite simple. Radio waves travelling in air reach the antenna and cause potential difference across its length. The RF-DC integrated circuits capture energy from the movement of those charge carriers. The energy can be stored temporarily in a capacitor, which can then be delivered across the load.
An RF-DC converter circuit generally consists of an antenna that captures RF signals, a matching circuit that matches the antenna impedance with the receiver for maximum power transfer, a voltage multiplier circuit that boosts the input voltage to a compatible value, and power conditioning circuits like low-pass filters that ensure smooth output voltage.
Recently launched power management ICs by e-peas feature an innovative buck-boost architecture that helps devices to deal with low voltages. It targets RF-harvesting applications. It can harvest energies as low as 275mV/3µW from the environment.
Researchers at the National University of Singapore and Tohoku University have discovered a way to use magnetic tunneling junctions (MTJs) for harvesting 2.4GHz RF energy. The MTJs consist of two magnetic materials separated by around 1nm. These junctions act as a high frequency oscillator when a voltage bias is applied. With this, the researchers were able to harvest 850nW of power without any bias, which is enough to power a red LED via an LTC3108 DC-DC converter.
Triboelectric nanogenerators
These devices are the best option if your design being worked on consists of a material where friction is involved, and 100 to 200µW of power is sufficient. The triboelectric generators harvest all sorts of wasted mechanical energy that is readily available to us due to actions such as walking, rotating tires, vibration, wind, flowing water, human movement, etc. These devices can also be used as self-powered sensors to detect changes arising from mechanical agitation.
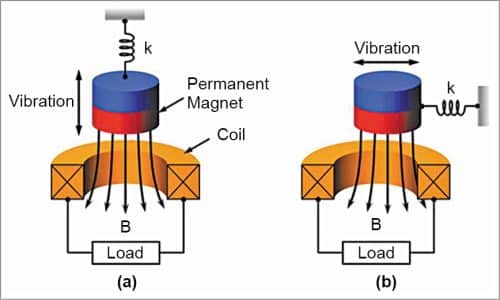
The working of these nanogenerators is relatively simple. When a mechanical stress is applied onto this device to bend it, the inner surfaces of the two sheets get into close contact and the charge transfer takes place. Due to electrical induction, one side of the surface attracts all positive charges while the other side attracts negative charges. This is called the triboelectric effect. When the stress is released, the surfaces separate and the opposite triboelectric charges generate an electric field in between, inducing a potential difference across the top and bottom electrodes.
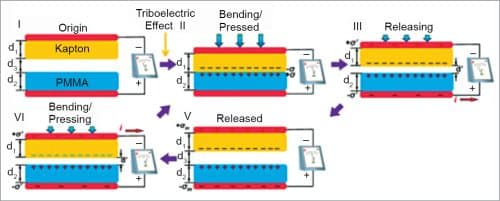
Thermoelectric harvesters
Thermoelectricity refers to the direct conversion of heat into electricity and vice versa. Applications that are being worked on to utilise the thermoelectric harvesters involve environments that contain temperature gradients and heat flow. These are solid-state devices that are heavily doped for high current generation. These harvesters can be employed in wearable devices for harvesting energy from body heat as well.
Recent studies suggest that transverse thermoelectricity can simplify energy harvesting from heat as the electric field and heat gradient are perpendicular. Researchers recently developed a crystal of rhenium and silicon that can produce electricity with notable efficiency at right angles to the heat flowing through it.
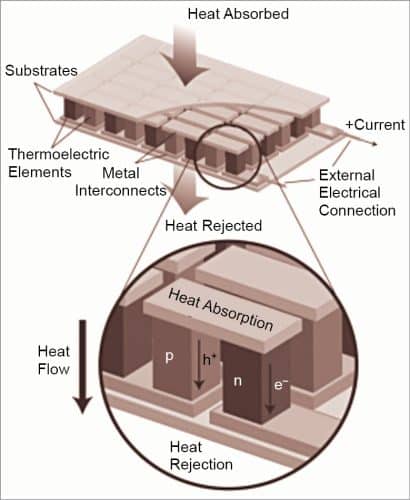
What to used where?
Summarising all the discussed harvesting techniques into three classes based on their feasibility, we can say the first class of systems work only when radiation coming from an on-board antenna is used. These are feasible for RF and microwave systems, but also harness ambient RF signals. An example of such a system would be wireless information and energy transfer (WIET) like the passive RFID backscatter or 6G communications at terahertz far infrared.
We can categorise the second class of systems as self-powered systems that rely on static electricity (like triboelectric generators) or useful chemical reactions. These systems can be commonly employed in wearables, or other such applications.
The third class is of systems that rely on mechanical energy. This includes vibrational energy harvesters, electrodynamic energy harvesters, and others. Vibrational energy harvesters can be integrated in various wireless systems and electrodynamic energy harvesters are mostly employed in portable electronic devices like radio, flashlights, phone chargers, etc.
Table 1 gives an idea of which harvesting technique is best advised for a certain application.
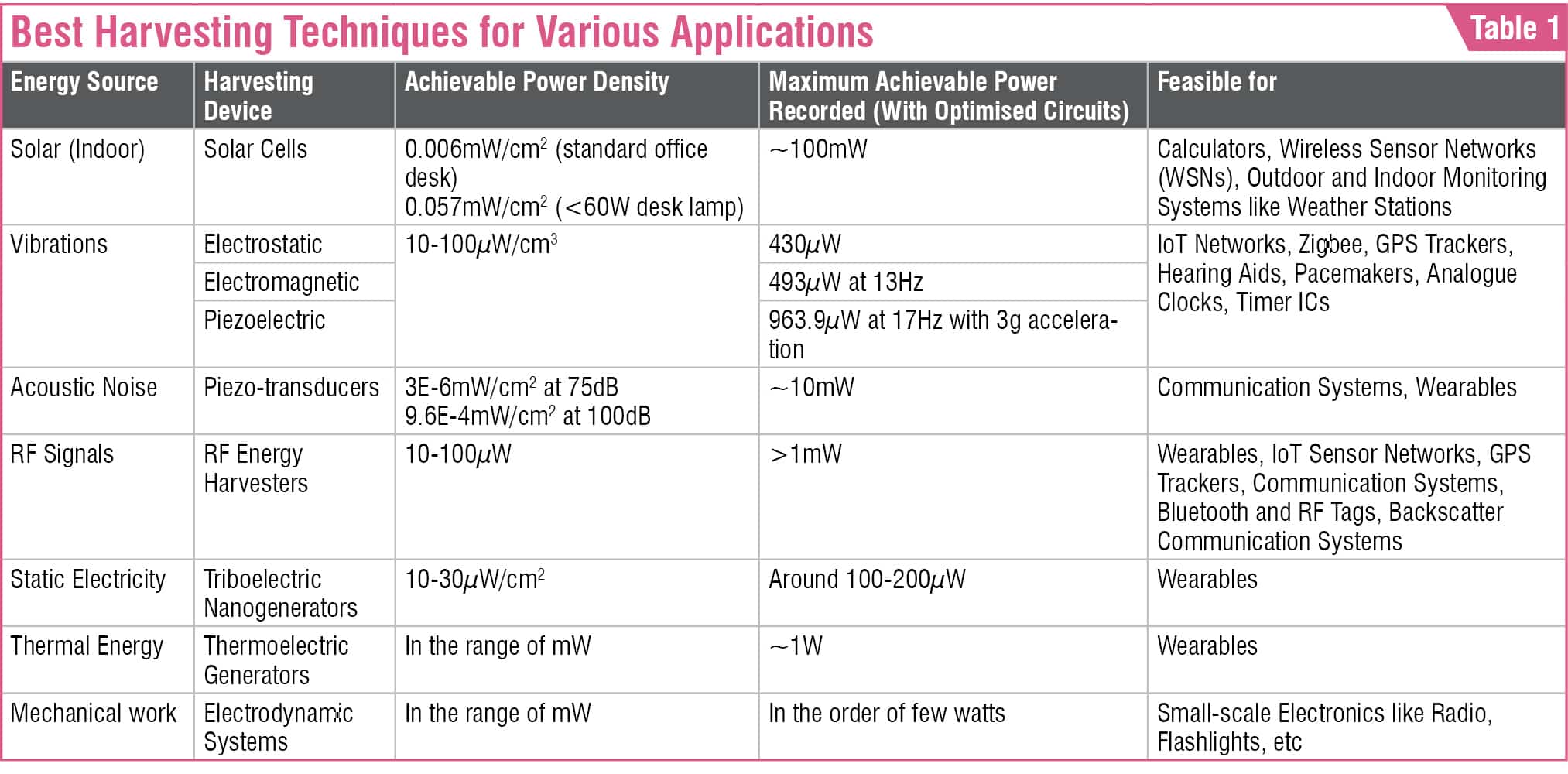
Implemented battery-less solutions
Now that the popular energy harvesting techniques are familiar, it is important to identify the reason for which these can be utilised for diverse applications. To get an idea about that, we learn from some of the most innovative products and technologies that employ energy harvesting techniques, eliminating the use of batteries.
Among all the techniques discussed, RF energy harvesting is most widely used. A fabless IoT company, Wiliot, in 2021 released its Bluetooth tag for easy tracking of shipments such as food and medicine. The IoT device can harvest energy from ambient RF signals and convert it into electricity for powering circuits. This tag allows customers to see all aspects of the item’s journey, for example, inclusive of the freshness of food.
The RF harvester, in this case, supplies an ARM microcontroller with a 3-core CPU, RAM, and ROM. The device uses three looped antennas for harvesting more energy and also takes advantage of the nanowatt power consumption of the ARM CPU during sleep mode. However, for power hungry devices, either the energy harvested should be increased or a reduction should be done in the circuit’s power consumption. If both these factors are looked into, the system can be highly reliable.
Coming to vibrational energy harvesters, researchers at MIT have developed a method for underwater communication that utilises piezo elements without any power source. The system utilises a master/slave model where the master has a power source that transmits acoustic signals, and the slave device detects the wave and uses its piezo transducers to generate power from the acoustic signal.
Thermoelectric and triboelectric harvesters are currently being employed in various wearable applications. Thermoelectric converters can be seen in smart watches and fitness trackers. Researchers are fabricating triboelectric generators inside fabrics, shoes, and other wearables for harvesting energy.
While choosing energy harvesters, the energy consumption of the application should be well predicted. There are solutions that help you build your platform or system from energy harvesters. For example, On Semiconductor’s RSL10 is a multi-sensor platform based on ultra-low-power solar cells. It integrates a low-profile 47µF storage capacitor onboard for energy storage. Its power consumption is just 55nW in standby mode and 10mW in Rx and Tx mode.
The research studies and innovative products given below are those that have emerged recently, showing the true potential of this battery-less technology and its consistent growth.
- Elimination of batteries by using wireless energy transmission is widely seen in Backscatter technology in RFID tags. The RFID reader has a power source which sends the signal. When the tag on the other end receives the energy via electromagnetic waves, its chip is activated when this energy travels through the tag’s internal antenna. The remaining energy is modulated with the chip’s data and flows back via the tag’s antenna to the reader’s antenna in the form of electromagnetic waves.
- To increase the safety of the patient, researchers at the Northwestern and George Washington Universities have developed a wireless, battery-free and fully implantable temporary pacemaker that dissolves when no longer needed. The pacemaker harvests energy from external near-field antennas, similar to the RFID technology. This wireless power transfer eliminates any leads that can introduce infections.
- Yet another wireless implantable device is an optoelectronic device for spinal optogenics (technique that involves the use of light to control neurons). It consists of a double-layer, rectangular-shaped magnetic coil antenna connected to a microscale inorganic light-emitting diode that can be implanted near the spinal cord for effective simulation of light-sensitive proteins.
- Researchers from the University of Massachusetts Amherst have created a unique solution that can harvest energy from ambient humidity via green materials. The device called Air-Gen, invented in 2020, consists of thin films of protein-nanowires that absorb water vapour from the environment and, due to the conductivity and surface chemistry of the nanowires, creates electricity between the electrodes. The energy produced is enough to power micro- and nano-electronic devices that can interpret biologically relevant stimuli.
- Researchers at UC San Diego have developed a thin wearable device that can harvest energy from sweat. The wearable device can be wrapped around a fingertip like a band-aid. The padding of carbon foam electrodes absorbs sweat and converts it into electrical energy. The electrodes in the device are equipped with enzymes that trigger chemical reactions between lactate and oxygen molecules in sweat to generate electricity. Underneath the padding is a piezoelectric element that generates additional energy when pressed. This energy is stored inside an integrated capacitor.
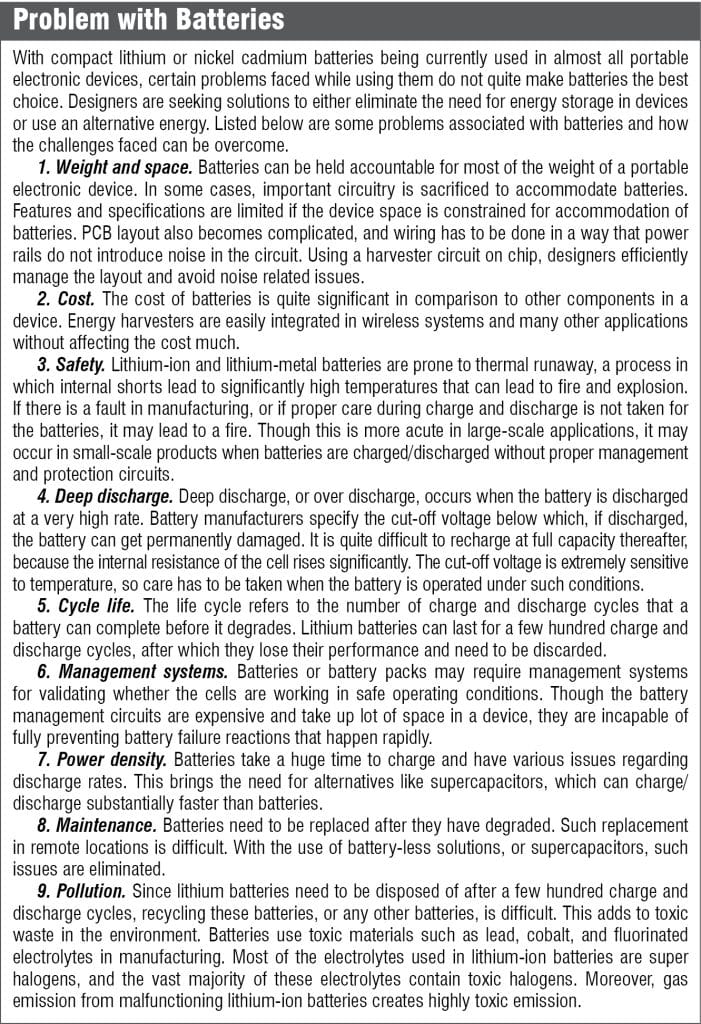
Supercapacitors – an alternative to batteries?
Unlike batteries, which rely on electrochemical reaction for charging, supercapacitors rely on electrostatic separation of charged particles in the electrolyte. They can be charged and discharged an infinite number of times until they start bulging.
So, supercapacitors do not require maintenance and are environment friendly, making them last longer as compared to the device they inhabit, while batteries do not. Hence supercapacitors are considered as alternative to batteries. Further advantages of supercapacitors are:
- Supercapacitors fully discharge with no damage. Capacity is all usable
- Can be charged and discharged faster
- They do not swell and shrink in use, and they tolerate ten times the power
- While charging, no special care is required
- They do not overcharge and overheat
However, supercapacitors come with their own challenges in terms of energy density, cost, size, and safety, as storing energy is potentially dangerous. But makers are phasing out toxic and flammable chemicals that have been used in supercapacitors, and now they have better safety records than lithium-ion batteries, according to IDTechEx.
Supercapacitors are practically being employed in many large-scale and small-scale applications as an alternative to batteries. A team of electrical engineers at the University of Rochester has developed a supercapacitor based solar harvester for computational systems. The system consists of a microcontroller-driven SEPIC converter that charges supercapacitors and targets embedded system applications requiring power in the 1-10W range.
Talking about the large-scale applications of supercapacitors, Shanghai Aowei buses made by Sinautec Automobile Technologies are fully based on ultracapacitors. These buses rely on supercapacitors for traction, having up to four times the life and greater reliability, though they need top-up charging after every few bus stops.
Conclusion
Looking at the current scenario, most wireless solutions are both battery-free and compact in size. Battery-less solutions, in addition to cost and size benefits, increase safety and reliability. Researchers and R&D engineers across the globe are trying to optimise energy harvesting circuits for more efficiency. Therefore, battery-free solutions will have a big impact on IoT devices, 6G communication systems, and wearables. It is to be seen when this technology will begin to solve major problems of physical batteries.
Darshil Patel is a technology enthusiast with a deep interest in Power Electronics