Electronic senses offer advantages of consistency as well as not having to use humans for what may sometimes be unpleasant or even dangerous tasks.
Electronics development has progressed well to replicate the human body’s five basis senses with electronic devices called e-skin, e-tongue, e-nose, hearing aids and electronic eye. In fact, electronics has moved further to sixth and seventh senses with wearable interaction and gesture control of electronic devices.
Moving further into the digital world, we will be able to induce not just familiar but completely new, never-before-known senses. For example, a banking chip implanted under the skin may, one day, create the sense of a full or empty bank account. Connected to the social network, we will experience ‘physically’ the distance to those speaking, or the actuality and the time-freshness of what will be said.
The first bunch of digital senses will simulate natural ones, just because we have to rest on something familiar in our cognitive experience. Then, we will develop derivative senses; these derivations will detach farther and farther from what we have been familiar with in our previous physical experience.
Once in the future, we will have moved far enough to become completely resettled in the digital environment that will turn out to be the natural environment for the new, digital post-human being. By that moment, we will be able to design completely new sensations with whatever range of excitement.
Basics
In electronics, sense is a technique used in power supplies to produce the correct voltage for a load. Although simple batteries naturally maintain a steady voltage (except in cases of large internal impedance), a power supply must use a feedback system to make adjustments based on the difference between its intended output and actual output. If this system is working, the actual output will be very close to the intended output.
Two types of senses are used depending on where the power supply output is measured. In local sense, the supply simply measures the voltage at its output terminals, where the leads connect them to the load. This method has the problem of not accounting for the voltage drop due to resistance of the leads, which is proportional to the amount of current drawn by the load. That is, the supply might be producing the correct voltage at its output terminals, but there will be a lower voltage at the input terminals of the load.
When this might cause a problem, remote sense can be used to force the power supply to counteract the voltage drop by raising the voltage at its output terminals. If successful, it will exactly cancel the drop along the leads, yielding the correct voltage at the input terminals of the load. This is accomplished by using separate sense leads, which are connected to the load’s input terminals, to measure the output voltage. (Because the sensing function draws only a very small amount of current, there is practically no additional voltage drop due to the sense leads themselves.)
Many power supplies that are equipped with remote sense can cause catastrophic damage to the loads if these turn on while the sense leads are unconnected. To avoid this, some supplies are equipped with auto sense, which automatically switches between local and remote sensing depending on whether the sense leads are correctly connected.
e-noses and e-tongues, as their names suggest, are inspired by the neurophysiology of smell and taste, and attempt to mimic the abilities of their human counterparts. These technologies automate evaluation of samples with complex composition and are able to recognise specific properties and characteristics.
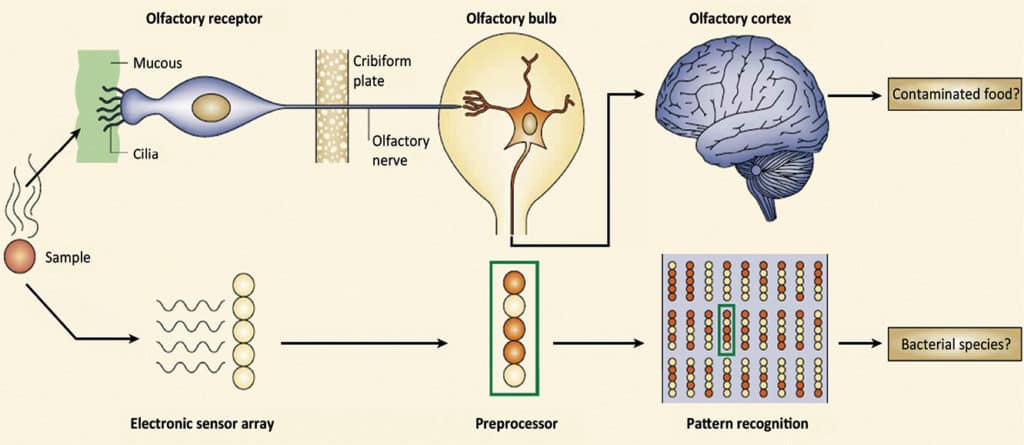
In animals, sensory information is processed by the neural system. Likewise, data collected through selective sensor arrays must be analysed by pattern recognition tools that employ various mathematical and statistical processing techniques. Such systems can provide quantitative results and, in some cases, are even able to detect differences that a human sensory panel cannot distinguish.
In food analysis, arrays of gas sensors are termed e-noses, while arrays of liquid sensors are referred to as e-tongues. The first scientific literature on these systems appeared in the 1980s but it has only been in the last decade, that special attention has been given to emerging technologies in electronic senses due to the food industry’s progressive interest in rapid at- and on-line analysis of product quality and safety.
Typically, e-nose instruments use four types of sensors: conducting polymers, metal-oxide semiconductors (MOS), metal-oxide semiconducting field-effect transistors (MOSFETs) and oscillating sensors such as quartz-crystal microbalances.
e-tongue instruments generally use the following analytical solutions: mass sensors, which are miniaturised solid-state devices that exploit piezoelectric effect; potentiometric methods, for example, ion-selective electrodes; and voltammetric or optical sensors, in which an indicator molecule changes its optical properties when exposed to a target analyte. Hybrid e-tongues, based on a combination of potentiometry, voltammetry and conductimetry, offer great potential.
Industrial applications
Electronic senses offer advantages of consistency as well as not having to use humans for what may sometimes be unpleasant or even dangerous tasks.Up to now, electronic senses have had straight industrial applications. For instance, food, beverage, pharmaceuticals, plastics, packaging and environmental industries have clear uses for electronic senses.
In food and beverage industry, e-noses can detect when flavours or odours in food are ‘off,’ and if there has been contamination or migration of compounds from plastic packaging into content. MOS odour sensors can also control how fast a flavour is diluted, ensure quality control or reverse-engineer an aroma. Electronic tongues can assess things like bitterness, taste stability over time or product adulteration.
Concrete applications of e-noses can be found in the analysis of meat flavours, volatile organic compounds (VOCs) formed during post-harvest ripening of fruits, ham product evolution during storage and olive oil defects, as well as identification of geographical origin of foodstuffs.
In the last decade, for example, Barilla has successfully implemented MOS-based e-noses in different quality control labs to recognise residual solvents and to continuously monitor the various plastic food-packaging materials adopted within bakery production sites. At Barilla, e-noses are, in fact, used as the first appraiser of packaging quality, which limits the number of gas chromatographic confirmatory analyses solely to the samples marked as uncertain or bad by the MOS instrument.
e-tongues can be used to monitor and discriminate among mineral water, coffee and soft drink samples. Reported applications of e-tongues in food analysis cover process monitoring, foodstuff recognition/characterisation, evaluation of ‘freshness,’ quality control and authenticity assessments.
Electronic senses were applied to evaluate the effect of brewing temperature on sensorial properties of espresso coffees (ECs) produced by a bar machine that was able to work with constant, increasing and decreasing water temperature profiles. The ECs were analysed by e-nose, e-tongue and e-eye to depict their aroma and taste fingerprint, and to evaluate the visual characteristics of foam. Physicochemical analyses were carried out to determine the extraction rate of typical EC components and evaluate their antioxidant activity.
Electronic devices coupled with multivariate statistical analysis demonstrated a good ability to discriminate and characterise coffee samples on the basis of their sensorial properties in relation to the brewing temperature. According to these results, electronic senses can be applied to assess the influence of percolation parameters on the sensory attributes of ECs, thus resulting in useful tools for optimisation of processing conditions.
Sensory characteristics of food products are gaining importance in the international food research and industry to meet consumer needs. Food producers make efforts to improve the quality and offer a wide range of foods. Comparison with existing brands in sensory point-of-view and developing products in sync with consumer needs are essential for the successful new product launch.
Usually a trained sensory panel is used to evaluate product quality. However, the reliability of sensory results is highly dependent on panelists’ acuity and correct application of the sensory practice. Therefore sensory attributes measurement using instrumental methods is a desirable aim. A new concept has been introduced in the sensor development using several sensors of low selectivity simultaneously.
Scientists have devised a detector that can distinguish smells better than a service dog. This sensor can detect explosive vapours and identify them. Called electronic nose, the device is able to sense and recognise traces of almost all types of explosives, from saltpetre to hexogen (which cannot be detected by even the most advanced technology).
For individual use
In the very short-term future, sight, taste and smell sensors will be available to new industries for incorporation into their own products, or for individuals directly. So, refrigerators of the future may have sensors that detect when food is going bad. These might be connected to a food-management system that alerts the user, who can then check in order to make a grocery list.
Cars may have sensors that detect carbon dioxide. The level of carbon dioxide rises in a car when driving with the windows shut. That’s dangerous because carbon dioxide can make the driver sleepy. A sensor would alert the occupants to stop for fresh air.
Doctors in the future may test patients’ breath to detect the onset of diseases like diabetes or cancer. A new study shows that organic compounds in exhaled breath can indicate whether a person has lung cancer—as well as its stage—and distinguish cancer from chronic obstructive pulmonary disease.
Skin is probably one of the best wearable technologies that nature ever developed. Skin on our fingertips contains pressure sensors that produce voltage pulses when we touch things. The frequency at which these pulses are created gives the brain information about what we’re touching.
Prosthetic limbs could soon be covered with an electronic skin that mimics human touch. Scientists have developed a flexible plastic skin that can communicate a sense of pressure to brain cells. The plastic skin generates voltage pulses when pressure is applied. The higher the pressure, the closer the carbon nanotubes are pushed together. This increases the conductivity frequency of voltage pulses.Electronic skin is capable of generating the same frequency of voltage pulses as human skin—up to 200 hertz. Being digital means the system is low powered, which is also important since, to mimic human touch, prosthetic skin will need to have thousands of sensors in the space of a fingertip to feel properly.
Currently electronic skin can only detect static pressure rather than moving pressure (for example, a brushing action). It is hoped that further development of a range of different sensors within five years will give artificial skin the full range of features involved in touch, including vibration, texture and temperature.
For visually impaired people, devices have been developed that can transmit the spectrum of colours and lighting around, along with spatial orientation into a mouth cavity, using slight electric stimulation.
A special electric collar can charge dogs with electric shock when these start barking too much or run too far away. So, it brings up the dogs with a sense of attachment. The dog collar may start with a vibration to warn before punishment.
Sensory punishing devices have already been developed for people. These punish the wearer with a slight electric shock in case the wearer passes a deadline, smokes after having pledged to quit or breaks some other rules established by themselves. The device is designed to facilitate the fight against bad habits.
Scientists are developing a feel-space belt that allows the wearer to feel the Earth’s magnetic field and be oriented in the four winds, just like birds and bats. The belt just transforms the magnetic currents into vibrations that the body can easily perceive. In reality, the new sensation is just a cognitive effect induced by a physical impact on receptors of the old sense, which is tactility.
It is safe to say that this transition of meaning of one ‘sense’ via the other sense is symbolical. It requires an intermediary. Therefore it requires time and effort to recognise and learn the content of signals, while the real, natural senses are immediate for perception as these require no symbolic interpretation. We feel cold or water directly by sensors designed to feel such things.
Technological advancements
As mentioned earlier, e-nose is an artificial olfaction device with an array of chemical gas sensors, a sampling system and a pattern-classification algorithm to recognise, identify and compare gases, vapours or odours. Thus e-noses mimic the human olfactory system.
Apart from food quality detection, these devices have been successfully used in a wide variety of applications including wastewater management, measurement and detection of air and water pollution, healthcare and warfare. One of their strengths is that the data gathered can be interpreted without bias.
Use of nanomaterials in e-nose applications is gaining ground, and so is the capability to create sensors with ultra-high sensitivities and fast response (partly due to a smaller structure). Smaller sensor size allows integration into a larger number of devices. An attractive class of materials for functional nanodevices is metal-oxide semiconductors. These offer the advantages of simple operation, ease of fabrication and compatibility with microelectronic processing, as well as low cost and low power consumption.
As regards e-tongue, within a few years, researchers anticipate a machine that determines the precise chemical structure of food and why people like it. Digital taste buds will also help us to eat smarter and healthier.
E-tongues are used in liquid environments to classify contents of the liquid, identify the liquid itself or discriminate between samples. Most e-tongues are based either on potentiometric or amperometric sensors. Taste sensors have artificial polyvinyl chloride (PVC)/lipid membranes that interact with a target solution such as caffeinated beverages. Change in the potential of the lipid membrane is the sensor output or measurement. Investigating potential change results in measuring the taste provided by the output of the chemical substances. Multiple sensors of the sensor array provide this output to form a unique fingerprint.
Hearing systems are increasingly being trained by listening to sounds, detecting patterns and building models to decompose sounds. One of the most common applications for sensors in this segment is hearing aids. Digital advances have made today’s hearing aids smaller, smarter and easier to use. The most advanced hearing aids can interact with other devices, such as smartphones and digital music players, to deliver sounds directly and wirelessly to the listener.
Recent improvements are based on better microprocessors and noise-reduction software so that the hearing aid can be selective about the types of sound it amplifies, muffles or suppresses. Much of current research is focused on directionality and speech enhancement. Sound systems can employ digital-signal processing to automatically shift between two different types of microphones in order to pick up either a single speaker’s voice or sound coming from all around.Digital-speech enhancement can now increase the intensity and audibility of some segments of human speech. Research projects are underway to reduce the size and cost of hearing aids, improve their directional capabilities, and identify and amplify desired sounds such as a human voice while muting background noise.
Electronic-skin prototypes are stretchy, thin films that can sense temperature, pressure and even monitor blood oxygen or alcohol levels. But most of these devices are missing hair—a key feature of real skin that allows us to feel a wider range of conditions.
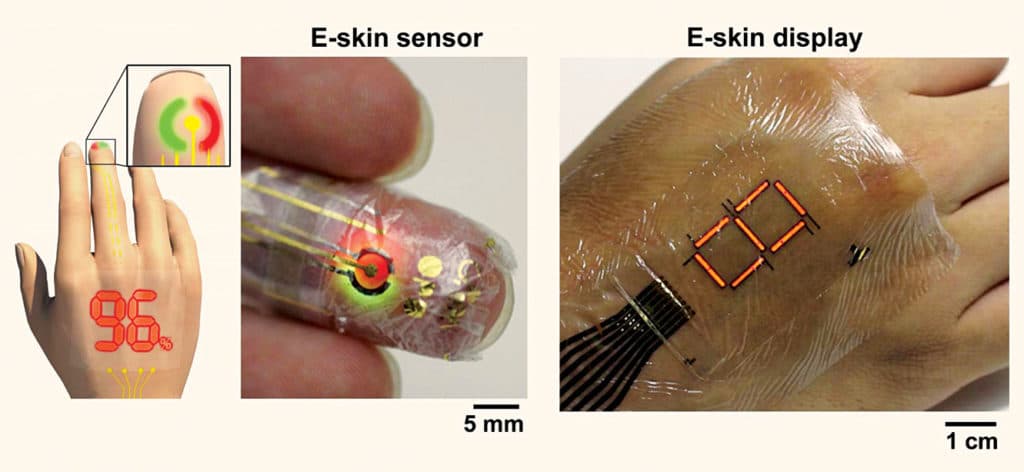
Now researchers have combined hair-like wires with electronic skin to make a more versatile sensor for robots, prosthetics and other applications. Robots and prosthetics are becoming ever more human-like, but the electronic skins designed to enhance their usefulness don’t yet have the full range of tactile senses that we have. To capture that sensation, some researchers have developed separate sensors that mimic the fine hair by sensing and detecting air flow.
Looking ahead
Thanks to significant advances in microfluidics and electronics, e-nose and e-tongue technologies will evolve both in terms of robustness and reduction in the current needed to optimise each application—a process that requires significant investment of time and resources. Miniaturisation will further extend flexibility.
Beyond food, e-sense technology may find applications in environmental analysis to detect water contamination or illicit drugs; in clinical diagnostics to monitor saliva, sweat or urine; and in agriculture to detect fungal contamination in feed. However, both e-nose and e-tongue technologies need improvements in sampling procedures (by reducing clean-up or extraction before analysis, for example) and reduction of carry-over/environmental noise (for instance, in the form of moisture contamination), which affect sensor drift and sensitivity.